How cryogenic microscopy could help strengthen food security
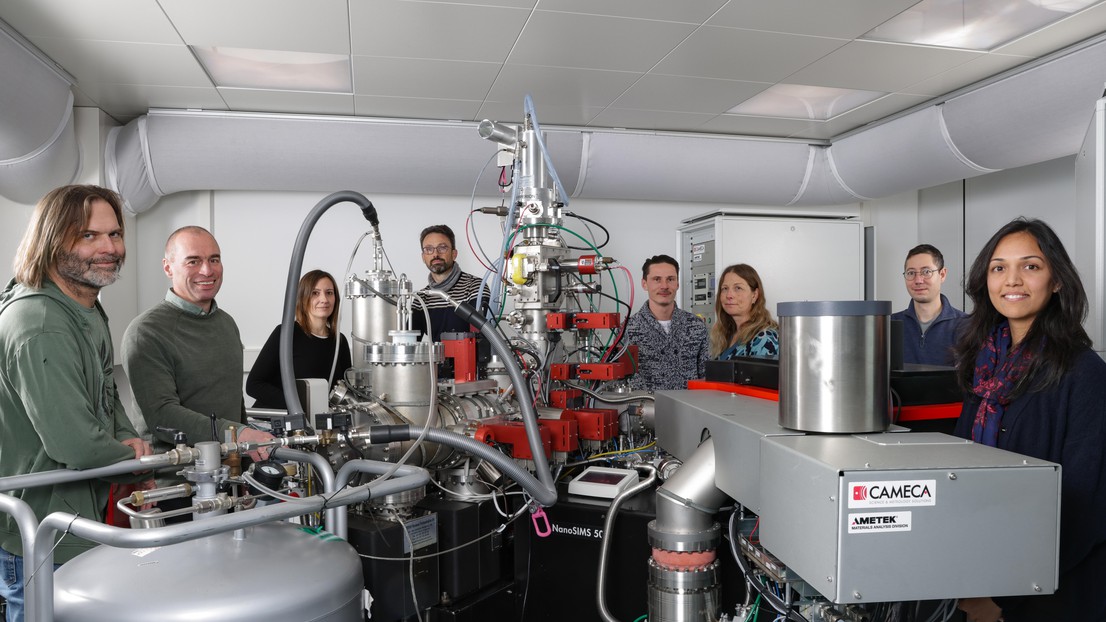
The research team around the CryoNanoSIMS instrument. © 2025 EPFL/Alain Herzog - CC-BY-SA 4.0
A joint EPFL and University of Lausanne research team reports on a novel observation of a plant protection mechanism in response to salt stress. The study opens new avenues of research to strengthen food security.
According to the United Nations, soil salinization affects between 20% and 40% of arable land globally, with human activity and climate change – especially rising sea levels – largely responsible for this process. While the human body needs sodium to function, this is not the case for most plants. In fact, excess salt around plants’ roots gradually blocks their access to water, stunting their growth, poisoning them and hastening their death. Ten million hectares of farmland are destroyed by soil salinization every year, posing a threat to global food security.

Scientists at EPFL and the University of Lausanne (UNIL) and their Spanish partners observed how ‘Salt Overly Sensitive 1’ (SOS1), a gene identified in 1996, protects the plant cells from salt. The team of biologists and engineers produced unprecedented images using the CryoNanoSIMS (Cryo Nanoscale Secondary Ion Mass Spectrometry) ion microprobe. With this cryogenic microscopy instrument – the only one of its kind in the world – they were able to obtain precise images of the location in which a specific nutrient is stored or used within a cell or tissue sample. Their observations show that, under high levels of salt stress, the ion transporter SOS1 no longer removes sodium but rather helps to load it into structures called the vacuoles within the cells. Better understanding this mechanism and working out why some species are more tolerant to sodium than others could, according to the scientists, allow us to develop new strategies to strengthen food security. Their findings have just been published in Nature.
We can now see where sodium is transported to at different levels of salt stress – something we were unable to do at this resolution before
First visual proof
“Our research provides the first visual proof, at the cellular scale, of how plants protect themselves against excess of sodium,” says Priya Ramakrishna, a postdoctoral researcher at EPFL’s Laboratory for Biological Geochemistry (LGB) and the lead author of the paper. “Previous hypotheses of this mechanism were based on indirect evidence. We can now see where sodium is transported to at different levels of salt stress – something we were unable to do at this resolution before.” The joint EPFL and UNIL team carried out observations in unprecedented detail with the recently developed CryoNanoSIMS instrument, that permits obtaining chemical images of biological tissue at a resolution of 100 nanometers, in this case on samples of plant roots that had been snap-frozen in a bath of liquid nitrogen and maintained at very low temperatures under vacuum, to preserve all elements in place in the tissue.
This approach allowed them to map individual plant cells and see where key elements, such as potassium, magnesium, calcium and sodium were stored in plant root tips – the part of the plant known as the “root apical meristem” – that contain the stem cells responsible for the development of the plant root system. The CryoNanoSIMS imaging showed the condition of the root at two different salt stress conditions.

A change of strategy
Under mild salt stress, the cells manage to keep sodium from entering. But the team observed a change of strategy under high salt stress: instead of evacuating the sodium, as previously thought, the SOS1 transporter helps to sequester it into vacuoles that serve to store unwanted products. “But this defense mechanism is energy-intensive, slowing down the plant’s growth, inhibiting its performance and ultimately leading to its death if the salt stress persists,” explains Ramakrishna. The researchers validated their observations by performing the same experiments on mutant samples lacking the SOS1 transporter gene, revealing its inability to transport sodium into the vacuoles, which explains its strongly increased sensitivity to salt. They also ran the tests using root samples taken from rice – the world’s most common crop – and found that, in this case too, the sodium was transported to the vacuole under high salt stress.
With this kind of truly interdisciplinary collaboration, we can match location with function and understand mechanisms and processes that have never been observed before
Matching location with function
For Ramakrishna, a plant biologist by training, the chemical imaging made possible by the CryoNanoSIMS instrument is a complete game changer. And the instrument could also be used to investigate how plants protect themselves against other threats, such as heavy metal pollution and microbes. “With this kind of truly interdisciplinary collaboration, i.e., blending biology and engineering, we can match location with function and understand mechanisms and processes that have never been observed before,” says corresponding author Anders Meibom, a professor at EPFL’s School of Architecture, Civil and Environmental Engineering (ENAC) and UNIL’s Faculty of Geosciences and Environment, in whose laboratory the CryoNanoSIMS instrument was developed.
Niko Geldner, the paper’s co-corresponding author, head of the research team at UNIL's Faculty of Biology and Medicine and leader of the UNIL team, is equally enthusiastic about this collaboration: “Plants are fundamentally dependent on extracting mineral nutrients from the soil, but we were never able to observe their transport and accumulation at sufficient resolution. The CryoNanoSIMS technology finally achieves this and promises to transform our understanding of plant nutrition, beyond the problem of salt.” Professor Christel Genoud, co-author of the paper and Director of the Dubochet Center for Imaging adds: “This technique is opening up an entirely new horizon in the imaging of biological tissue and places our institutions as leaders on this frontier”.

Priya Ramakrishna, Francisco M. Gámez-Arjona, Etienne Bellani, Cristina Martin-Olmos, Stephane Escrig, Damien De Bellis, Anna De Luca, José M Pardo, Francisco J. Quintero, Christel Genoud, Clara Sanchez Rodriguez, Niko Geldner and Anders Meibom, “SOS1-dependent vacuolar sodium accumulation revealed by elemental cryo-imaging”, Nature, 15 January 2025. DOI : 10.1038/s41586-024-08403-y